UW scientists and engineers share their thoughts on the potential of quantum research and why it’s so important now.
Image by Gerd Altmann / Pixabay
Scientists and engineers across the University of Washington are pursuing quantum research projects spanning from creating materials with never-before-seen physical properties to studying the “quantum bits” — or qubits (pronounced “kyu-bits”) — that make quantum computing possible. Here, three UW scientists and engineers share their thoughts on the potential of quantum research and why it’s so important now.
Nancy Allbritton speaks at a College of Engineering event in 2023.
Nancy Allbritton
Frank and Julie Jungers Dean of the College of Engineering
Related story
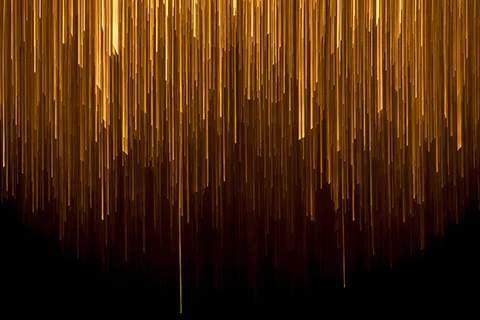
Accelerating a quantum future
Learn how College of Engineering and College of Arts & Sciences researchers are helping to establish the UW as a global leader of the coming quantum age.
Why is quantum such a big deal?
Some readers may remember when the personal computer and classical computing emerged in the 1980s. Those technologies changed nearly everything. Recent advances in quantum research promise breakthroughs that will also fundamentally change how we live and work. Thanks to quantum information science, we’re advancing technologies like GPS, MRI scans and lasers used in surgery. It can help us address major societal issues, and it promises economic benefit.
In recent years, the U.S. government has identified quantum as a key technology investment area. Congress signed the National Quantum Initiative Act into law in 2018, and the CHIPS and Science Act of 2022 boosted federal quantum investment. In 2023,U.S. Secretary of Commerce Gina Raimondo called on universities to triple their graduation of new engineers over the next decade as part of the long-term vision for America’s technological leadership.
This is a call to unite behind a shared objective, think boldly and mobilize public-private partnerships. I believe that because of its strong ties between research universities and industry, the United States — and the state of Washington in particular — is poised to be a quantum leader.
What are the challenges?
Competition for talent and funding is aggressive, and governments and industries worldwide are making big investments to lead in this area. Only a few universities across the globe have quantum programs, but that is quickly changing.
Another big issue is the lack of a prepared quantum workforce. Companies already have a difficult time filling skilled STEM jobs, and this is further complicated when jobs require advanced degrees in physics, chemistry, materials science, engineering and computer science. Universities, governments and private companies need to partner to expand the number of quantum-trained professionals. In Washington, workforce development will have a major impact on established companies like Microsoft, Boeing, Amazon, T-Mobile and Google, as well as on emerging start-ups and companies that don’t even exist yet.
How is the UW playing a role in advancing quantum?
The UW can provide leadership, research and workforce education. Thanks to support from the UW President and Provost, this institution has been intentionally growing its quantum information science and engineering programs across the College of Engineering and College of Arts & Sciences. It has strong roots in quantum discovery, too: not one but two UW scientists have received the Nobel Prize in Physics for quantum research — Hans Dehmelt in 1989 and David Thouless in 2016.
Today many of our quantum researchers are recognized as global leaders. Thanks to collaborations with industry partners — including Microsoft, Boeing, Google and Amazon — and with the Pacific Northwest National Laboratory (PNNL), they have been advancing quantum research and teaching.
Why is it important to invest in quantum research and education at the UW and in Washington?
We need to prepare students for jobs within quantum information science and engineering. And we need to support research that helps promote economic and national security while expanding the talent pool for current and future industries here in Washington.
The competition to develop “Quantum Valley” is heating up. It will require the sort of outlook and investment that paved the way for Silicon Valley in California. This won’t happen overnight, but we need to start building it now.
Kai-Mei Fu at work in the Quantum Defect Laboratory, which she directs at the UW. Photo by Dennis Wise / University of Washington
Kai-Mei Fu
Professor of physics and of electrical and computer engineering
QuantumX Co-Chair
Related story
Q&A: UW researcher discusses future of quantum research
UW Professor Kai-Mei Fu discusses the potential of quantum research, and why it’s so important.
What is “quantum?”
Originally, “quantum” just meant “discrete.” It referred to the observation that, at really small scales, something can exist only in discrete states. This is different from our everyday experiences. For example, if you start a car and then accelerate, the car “accesses” every speed. It can occupy any position. But when you get down to these really small systems — unusually small — you start to see that every “position” may not be accessible. Similarly, every speed or energy state may not be accessible. Things are “quantized” at this level.
That’s not the only weird thing: At this small scale, not only do things exist in discrete states, but it’s possible for things to exist in a combination of two or more different states at once. This is called “superposition.”
How is superposition useful in developing quantum technology?
Let’s take quantum computing. In the information age of today, a computational “bit” can only exist in one of two possible states: 0 and 1. But with superposition, you could have a qubit that can exist in two different states at the same time. It’s not just that you don’t know which state it’s in. It really is coexisting in two different states. Thus, it’s possible to compute with many states, in fact exponentially many states, simultaneously. The power is in being able to control that superposition.
What are some advancements that could stem from controlling superposition?
There are four main areas of excitement. My favorite is probably quantum computation. We know that the power of quantum computation will be immense because superposition is scalable. This means that you would have much more computational space to utilize, and you could perform computations that our classical computers would need the age of the universe to perform. So, we know that there’s a lot of power in quantum computing. But there’s also a lot of speculation in this field, and questions about how you can harness that power.
Besides quantum computing, what other applications are there?
Another area is sensing for more precise measurements. For my research, I work with atoms arranged into a perfect crystal and then I create “defects” by adding different atoms or taking out one atom in the lattice. The defect acts like an artificial atom and it will react to tiny changes nearby. These changes are normally so small that they would be hard to measure at room temperature, but the artificial atom amplifies the changes into something I can see. Some crystals will radiate light when I shine a laser on them. By measuring that light, I can detect a change. I get excited because we know that all these things are possible in theory, but we’ve just hit the timescale where we’re starting to see real technological applications.
Another area is quantum simulation. There are many potential applications in this field, such as studying new energy storage systems or making an enzyme better at nitrogen fixation. Essentially these problems require making new materials and are too hard for classical computers to simulate or predict. But quantum simulation could, using a quantum computer.
The final area is quantum communication. When you’re transmitting sensitive information, you can create a key to encrypt it. With quantum encryption you can distribute a key so fundamentally secure that if you have an eavesdropper, they leave a “mark” that you can detect.
What’s happening in terms of quantum education?
We’re crafting a common framework and language for quantum education. Quantum involves many fields, including chemistry, computer science, material science, chemical engineering and theoretical physics. Historically these fields have had their own approach and vocabulary. At the UW, we’ve launched a core curriculum in quantum for graduate students who want to pursue careers in this field. Through the Northwest Quantum Nexus, we also have partners for internships. And while we don’t currently have a quantum computer on campus, we are gathering materials to construct a quantum processor — the basis of a quantum computer — as part of our educational curriculum.
We need more scientists in quantum. There are too many questions to answer, and every field in quantum is growing. It’s going to change how we approach problems — in communication, in software, in medicine and in materials. It will be beyond what we can think about today.
Charles Marcus adjusts equipment at the Niels Bohr Institute in Copenhagen, Denmark. Photo by Ola Joensen / Niels Bohr Institute
Charles Marcus
Professor of materials science and engineering and of physics
Related story
Asking quantum questions
Asking deep – possibly unknowable – questions has been the driving force behind professor Charles Marcus’s entire life.
Why are we hearing so much about quantum now?
Two important things have happened in the last 10 years. One is that experimental control over quantum mechanics systems has emerged and become highly developed. In other words, how to “do” quantum research has become more feasible. The other is that progress has been made in terms of how to use quantum computers to solve important societal programs. For a long time, people didn’t know how to use these machines, but that’s changed.
It’s generally uncommon to develop new technology and its applications simultaneously. For example, we developed lasers then figured out how to use them. With flight, we established a need then came up with the technology to achieve it. With quantum, these have co-evolved over the last two decades. The last decade especially has seen a big influx in participants and in the idea that the frontier is expanding across all areas of quantum, not just computing.
What’s happening at the UW that makes it an exciting time to be here?
It is a remarkably exciting time! Over the last few years, the UW has hired several early and mid-career faculty across several disciplines—electrical and computer engineering, materials science and engineering, physics and more. And the UW is encouraging and supporting collaborative quantum research across disciplines. Quantum research doesn’t reside in one department or college but across several. This is a major strength, and it means that something catalytic can occur.
You’ve led quantum research at several organizations, including Harvard University, Microsoft and the University of Copenhagen. What are you most looking forward to now that you’ve joined the UW faculty?
Honestly, there’s no place else I’d rather be. I am eager to work as part of the growing cluster of quantum researchers at the UW. Cooperation over competition was very much a part of the culture at the Niels Bohr Institute, where I taught while at the University of Copenhagen. There, researchers work together to advance a common interest. I want to help encourage and expand a similar approach to collaboration here. I’m also excited to shepherd exchange between the UW and University of Copenhagen — in terms of research collaboration, equipment sharing and more.
When it comes to quantum, why is it critical for academia, industry and government to be in sync?
The quantum field is in its infancy. As it develops it will require a big shift in how we think about innovation, which has traditionally followed a linear path: Government funds research, which takes place in academia and eventually is spun out and taken to market by industry.
With quantum, not only is it essential that an academic-industry-government relationship exist, but that all partners recognize it as cyclical. Some readers may remember the 1979 book Gödel, Escher, Bach: An Eternal Golden Braid, by Douglas Hofstadter. It gives us a good metaphor to apply here. With quantum, we can consider another type of braid: education, government and business — quantum must consistently be a braid of these that is always renewing and recursive, not driven toward a single, linear destination.
Why is it important that this work be situated in the Pacific Northwest?
This area has the right ratios of hard sciences, medical sciences, engineering and advanced information technology. It’s not Silicon Valley here, it’s something else. And the relationship between universities and business is strong here. There’s a lot of energy and a lot of seeds that have been planted — for example, by Kai-Mei Fu and others who’ve established QuantumX.
The real answer to what quantum computers can do is “no one knows.” But the Pacific Northwest is a high tech leader, and the interface between science and technology is strong. In many ways, the UW will be the engine driving this work.
Originally published May 8, 2023